ATP is required for monomer biosynthesis and the polymerization of these monomers into cellular
macromolecules (proteins, RNA, DNA). In the rumen, complete oxidation of feedstuffs is not
possible (anaerobic environment) and ATP yield (mol ATP/mol energy source catabolized) is
generally low. Bauchop and Eldsen (5) compared growth yields of several microorganisms with
the theoretical amount of ATP that would be available and coined the term YATP (g bacterial
DM/mol ATP). The overall average was 10.5 g cells/mol ATP, and for many years it was assumed
that YATP was fairly constant. The actual range was 8.3 to 12.6. Baldwin (4) applied this concept
to the rumen and suggested that the YATP values for the rumen were probably higher (average of
16.5) .
With the advent of continuous culture techniques, it became apparent that bacterial yield was
related to growth rate and that yields were lower at slower growth rates. These observations led to
the conclusion that bacteria used energy for "maintenance" as well as growth (42). The
"maintenance functions" of bacteria have not been precisely defined, but ion balance across the
cell membrane is probably most important (60). The overall concept of bacterial maintenance is
analogous to the maintenance energy of animals. Growth cannot occur until the maintenance
requirement is met, and maintenance makes up a larger fraction of total energy utilization when
growth rates are low. Thus, the yield value is dependent on the growth rate (k), the maintenance
energy of the microbes (m = g carbohydrate/g microbes/h), and the theoretical maximum growth
yield at a given maintenance requirement (Yg= g microbes/g carbohydrate). The following
equation integrates the various components composing microbial yield:
1/Y = m/k + 1/Yg
The maintenance energy of ruminal bacteria is less than that observed for many other bacteria (28,
51). However, average bacterial growth is low in the rumen, meaning maintenance can still be
significant. With a maintenance of 50 mg glucose/g bacteria/h (28), a theoretical maximum
growth yield of 40 g bacteria/100 g organic matter fermented, and growth rate of .05/h-1, 29% of
the total energy utilization would be devoted to maintenance.
In order to maximize microbial yield one first needs to identify the specific substrates required by
the several types of microorganisms that inhibit the rumen eco-system. Table 1
(Click here to view Table 1). identifies several
species of microorganisms and the substrates they are most likely to hydrolyze and/or ferment.
Although microorganisms are generally categorized as cellulolytics or amylolytics, many of the
rumen bacteria can ferment both structural and non-structural carbohydrate and produce a variety
of end products of fermentation.
The type, amount, and rumen availability of a given substrate (feedstuffs) will establish an
environment more conducive to a given species of microorganisms. However, because energy
sources are often the limiting factor to growth of individual species, several depend on being able
to efficiently use a variety of substrates. An example of this diversified approach would include:
Bacteriodis amylophilus and many of the ruminococci which can use starch and cellulose, but not
glucose and other sugars (52). Some bacteria have become very specialized. For example, rumen
cellulolytic bacteria have an obligate requirement for branch chain amino acids and depend on
other bacteria to supply branch chain volatile fatty acids to synthesize their branch chain amino
acids. Although this approach decreases the needs for specific carbon and/or energy sources, it
increases the species dependency on other bacteria.
Energy source is a key limiting factor for growth of individual species of rumen bacteria. The
diversity and efficiency of carbohydrate utilization can significantly effect growth rate.
In the rumen, nutrients are plentiful soon after feeding. A fast growth rate enables a more rapid
conversion of nutrients into microbial dry matter. Several hours after feeding, when nutrients
become less available, growth rates are also decreased (52).
Dynamic Factors Influencing
Rumen Starch Degradability and Postruminal Carbohydrate Delivery
The ruminal digestion process is a dynamic sequence and synergy of events that influences
fermentation end product and delivery of carbohydrate sources postruminally. The degree to which
these processes are manifested in the system is highly dependent on the type and amount of
carbohydrate sources consumed by the animal. Rate and extent of digestion are critical
components in the process. Table 2 (Click here to view Table 2).
shows different carbohydrate sources, rumen starch
digestibilities, and escape values.
Grain Processing. The effect of processing on
utilization of the starch of cereal grains has been reviewed extensively (16, 61). Generally,
processing is associated with increased efficiency of nutrient utilization by ruminal
microorganisms and in the total tract. The term processing can be subdivided into physical and
chemical methodologies. Physical processing methods usually consist of breaking, cracking,
grinding, rolling, or pelleting grains. Physicochemical modifications involve the application of
heat and water, which act to hydrate and swell amorphous and crystalline structures of the starch
granule. This alteration in structure enhances amylolytic digestion by both microorganisms and
pancreatic enzyme action. The degree of moist heat application to grain, in addition to physically
reducing particle size, has benefits greater than either process alone (61). Chemical treatments
have been shown to enhance or retard ruminal degradation depending on the chemical and its
concentration used.
Decreasing corn flake density resulted in a linear decrease in ruminal pH and an increase in
postruminal and total tract digestibilities (69). Galyean et al. (15) further indicated that processed
corn (steamflaking and high moisture ensiling) resulted in increased starch digestibility beyond
that of reducing particle size alone. However, grinding was more beneficial in enhancing DM
digestion of unprocessed compared with processed corn. Nocek (36) showed that high moisture
shelled and ear corn had a greater extent of ruminal digestion than dry forms and that grinding
generally increased the extent of degradation for all forms of corn. A similar effect was observed
with pelleting (58).
The native protein configuration of the kernel in relation to starch granules can influence starch
degradability. Starch granules can be embedded in a protein matrix of the endosperm of cereal
grains, especially in corn and sorghum (49). Processing can disrupt this matrix and allow starch to
be more accessible to enzymatic digestion. However, certain types of processing (those causing
gelatinization) may lead to formation of indigestible starch-protein complexes (62).
Most processing methods increase ruminal starch digestion, which usually increases percentage of
starch digestion in the small intestine as well (40). Under some situations, it may be beneficial to
increase the supply of starch to the lower gut. Although feeding of whole or coarsely cracked corn
decreased rumen starch digestibility, compared with more finely ground corn, the larger corn
particles may not be enzymatically digested in the small intestine. In addition, the passage of
larger, more dense corn particles from the intestinal tract is accelerated, which decreases total tract
digestion. Thus, the initial objective of shifting rather than limiting starch digestion may be
nullified (40).
Postruminal Carbohydrate
Delivery
The dynamics of postruminal starch digestion have received very little attention compared with the
rumen. However, the same rules apply: 1) feed particles must pass through the tract, 2) feed
particles must be reduced in size or digested, and 3) end products of digestion must be absorbed.
Major differences from the rumen are that mammalian enzymatic digestion predominates, and
substrate uptake for the host is primarily through active rather than passive transport. These events
are dependent on the amount of starch and the rate at which it is delivered.
The relationships among intake, site of digestion, and extent of starch digestion in dairy cattle
were examined by combining the results of 13 production studies (6, 7, 11, 17, 24, 25, 30, 32, 39,
43, 48, 55, 57). All studies utilized cows in the first half of lactation, having as the primary
objective to compare NSC sources.
Total starch intake (kg/d) was correlated positively to intake (kg/d) of ruminal degradable starch
(RDS), ruminal escape starch, total tract digestible starch, and intestinal digestible starch (IDS).
Rumen degradable starch and rumen degradable carbohydrate [calculated by Nocek and Russell
(37)] were correlated positively, as expected. However, RDS only accounted for about 60% of the
variation associated with the rumen degradable carbohydrate component, indicating that structural
carbohydrate digestion can contribute a large portion of total fermentable carbohydrate. Ruminally
and intestinally digestible starch were correlated in a positive fashion. Ruminal and total tract
digestibility of starch were highly related. Also, IDS and total tract digestibility were related
positively. Although this relationship was not as strong as that between RDS and total tract
digestible starch, total tract digestible starch is influenced to a greater degree by ruminal rather
than by postruminal digestibility.
The relationship between starch escaping the rumen and intestinal digestion was evaluated to
determine whether postruminal starch digestion was limiting. There was a positive relationship,
indicating that, as ruminal escape starch increased, intestinal digestion also increased (Figure 1).
No quadratic or cubic components were identified in this relationship. It must be borne in mind
that intestinal digestion represented both small and large intestines. These data compare with
those of Owens et al. (40), who demonstrated a similar effect in a summary of beef cattle data.
Although more starch is digested postruminally as intake is increased, efficiency of starch
digestion suffers. Starch entering the small intestine (percentage of intake) was plotted against
intestinal starch digestion (percentage entering) (Figure 2). As starch delivery increased, starch
digestion as a percentage of that entering the small intestine decreased. The relationship coincides
with data demonstrating decreased energy digestibility associated with increased intake above
maintenance (10, 33).
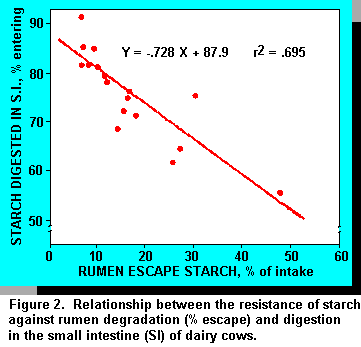
Small Intestinal Carbohydrate Uptake and
Glucose Absorption. Glucose uptake by the mammary gland is the major determinant or rate
of milk secretion (27). Mammary glucose uptake is a linear function of glucose availability to the
gland, as is the relationship between milk production and plasma glucose concentration (26, 27).
Kronfeld et al. (28) calculated that 72g of glucose uptake by the mammary gland were required to
produce 1 kg of milk. Thus, a cow producing 40 kg of milk requires about 2.9 kg/d of glucose
uptake by the mammary gland. With this estimated requirement for glucose, dietary manipulation
to enhance supply of glucose to the mammary gland may be a useful strategy to increase
production performance.
In the ruminant, logical modes of increasing plasma glucose are through providing rumen
fermentable carbohydrates that result in glycogenic substrates for the liver and starch sources that
escape ruminal fermentation. These approaches should result in a net positive glucose appearance
at the portal-drained viscera (PDV). In theory, the latter should be an energetically efficient means
to provide increased circulating glucose (less heat loss due to fermentation), providing that
ruminally available carbohydrate does not limit microbial protein synthesis. The way in which the
small intestine digests and absorbs starch and the resultant glucose available for mammary uptake
is influenced by several factors: 1) intestinal carbohydrase and pancreatic amylase activities and
starch hydrolysis, 2) visceral metabolism of glucose, and 3) regulation of endogenous glucose.
Although postruminal provision of starch seems like a logical mode of bypassing the inefficiencies
of the rumen and enhancing systemic glucose supply, there is some question as to what proportion
of starch entering the small intestine actually is absorbed as glucose and, once absorbed, what its
metabolic fate is upon delivery to the hepatic portal vein.
Most studies in dairy cattle have been limited to in vivo measurements to determine starch
disappearance from the gastrointestinal tract. These studies do not consider the cost of metabolism
by gut tissue, which ultimately influences net presentation of nutrients (net absorption) to the liver
(portal vein) and other tissues (muscle and mammary gland) (27, 47).
Lactate carbon can be converted to glucose and other metabolites in the liver (44). However,
lactate carbon accounts for only 12 to 15% of whole body glucose (2, 63).
Acetate utilization by PDV in sheep (41) is associated primarily with oxidative metabolism rather
than incorporation into mesenteric fat and represents about 25% of the whole body acetate
turnover on alfalfa-based diets. Propionate is derived primarily from gut absorption and shows a
net positive absorption in PDV. Hepatic uptake is associated primarily with glycogenesis.
In lactating cows (4 and 8 wk postpartum), net PDV glucose flux was negative and not influenced
by week postpartum (46). This negative flux was a result of greater use of arterial glucose by PDV
rather than absorption from the gut into portal blood (22). These cows were fed diets consisting of
60% corn silage and 40% concentrate. A higher level of concentrate, as may be appropriate in this
stage of lactation, may have resulted in a greater net flux of PDV glucose. Nonetheless, net hepatic
glucose production (3.1 kg/d) more than adequately met mammary (2.3 kg/d) and other glucose
requirements of cows producing 32 kg of milk (45).
There appears to be a threshold at which a net glucose appearance at the portal vein is observed.
This seems to be evident either when glucose is infused or when intakes are high, both of which
promote increased starch or DEI and rumen bypass of starch. Density and intake of metabolizable
energy also dictate the relative glucose needed to support metabolism of PDV. It seems this
requirement must first be met prior to net positive absorption of glucose at the portal vein.
Possible explanations for the different relationship between DEI and PDV flux would be as
follows: positive flux would indicate that a threshold of glucose is needed for gut metabolism, and,
once met, a net positive portal appearance would occur. Negative flux would suggest that, as DEI
increases, there is a greater oxidative drain on glucose for gut metabolism to process and
metabolize nutrients (increased metabolic and secretory cell processes, protein turnover, cell
transport, etc.). However, there probably are not enough data to develop a sound relationship or
conclusion.
Relative Value of Carbohydrate
Sources
The primary value of carbohydrate is to provide substrate for microbial protein yield (absorbable
amino acids). Since microbial amino acids are excellent quality, their value in meeting host amino
acid requirements is critical. However, the differential in microbial amino acid provision and
requirement of the host must be met. Supplementing this differential is also a cost that needs to be
considered in valuing carbohydrate. Inherent in this process is the concept that carbohydrate
balance (NSC versus structural) and DIP must be optimized to maximize rumen health.
In order to characterize potential carbohydrate value, a few very simple concepts were used: 1)
rumen degradable starch drives microbial protein yield ,and 2) if processing is involved, there is a
cost to increase RDS per unit of dry matter. There are many sources of carbohydrate that could
provide varying amounts of RDS (Table 2).(Click here to view Table 2).
However, this exercise focussed on two primary
sources; barley and corn and the relative value added through processing these common
sources.
Cost of Rumen Degradable
Starch
Table 3 illustrates cost/kg DM for various processed forms of corn and barley. It is realized that
cost can vary considerably depending on several factors. However, the objective of this exercise
was to develop a relative comparison to illustrate the value of carbohydrate. The RDS values used
for this evaluation were from Nocek and Tamminga (38) and Corneau and Doreau (8). Cost/kg
DM increased as RDS increased. However, the relative value of providing RDS was variable
depending upon process. For example, finely ground corn and barley were two processes which
dramatically increase RDS for a minimal cost/kg. For corn, grinding increased RDS by 22% at a
cost of $.005/kg DM. Steamflaking corn increased RDS by 36.7% at a cost of $.025/kg DM. Fine
grinding barley increased RDS by 51.7% at a cost of $.005 kg/DM, whereas steamflaking
increased RDS by 48.5% at a cost of $.025 kg/DM.
Cost of Bacterial Protein from
RDS and UIP
The relative value of increased RDS is to provide additional substrate for bacterial growth.
Assuming a maximal growth rate of .4g bacteria per g of RDS, (37), cost/kg of metabolizable
protein from additional RDS due to processing (relative to whole corn or whole barley) was
calculated. It was assumed that bacteria contained an average of 6.25% crude protein and 60% of
protein is metabolizable protein. Figure 3 illustrates the relationship of cost/kg DM and
metabolizable protein from bacteria as a result of processing. As cost/kg DM increased, bacterial
metabolizable protein increased quite sharply initially. However, beyond $205/ton, there was no
further value to processing because no additional RDS could be derived to support microbial
protein yield.
%20of%20bacterial%20protein%20derivedfrom%20RDS%20as%20a%20result%20of%20processing%20and%20the%20cost%20of%20different%20UIP%20sources.%20Metabolizable%20proteinvalues%20for%20UIP%20sources%20were:%2068.0,%2057.0,%2051.2,%20and%2040%25%20for%20fish,%20meat%20and%20bone,%20corn%20gluten,%20andfeather%20meal,%20respectively.%20Grinding%20RDS%20sources,%20especially%20barley,%20clearly%20was%20the%20mosteconomic%20route%20to%20provide%20additional%20metabolizable%20protein%20beyond%20what%20whole%20corn%20and%20barleycould%20provide.%20On%20a%20relative%20basis,%20it%20is%20less%20economical%20to%20provide%20metabolizable%20protein%20fromtraditional%20high%20protein%20UIP%20sources%20compared%20to%20processing%20to%20obtain%20RDS,%20to%20a%20threshold%20whereadditional%20RDS%20will%20be%20of%20benefit%20in%20the%20total%20ration%20(i.e.%20%3c40%25%20NSC).%3cp%3e%3ccenter%3e%3cimg%20src=)
Figure 5 and 6 show the relative cost of furnishing metabolizable methionine and lysine. It was
assumed that bacterial metabolizable protein is 2.6% met and 8.2% lys. Regardless of method,
providing additional RDS through processing was still a better value for providing it through UIP
sources. Although we usually think of corn gluten meal as a good source of methionine,
metabolizable methionine is relatively low compared to fish meal and meat and bone meal. The
value of rumen protected amino acid sources such as Smartamine-M in providing metabolizable
met is very favorable to other sources. Likewise, bacterial protein is an excellent and cost effective
source of providing metabolizable lysine.

Summary
The significance of rumen available carbohydrate is to provide an optimal environment for
enhanced rumen health and substrate to maximize microbial protein yield. There appears to be no
limitations to intestinal starch digestion. However, the efficiency of use decreases with increased
passage to the small intestine. The provision of postruminal starch is primarily to support
oxidative metabolism and spares the endogenous synthesis of glucose and specific amino acids for
gut metabolism. It appears that after these requirements are met, postruminal provision of starch
may then appear as glucose in portal vein viscera and used for milk synthesis rather than oxidative
purposes. Processing significantly changes rumen availability of starch, however, at a cost.
Providing metabolizable protein from microbial synthesis derived from RDS is more cost efficient
than by supplementation with traditional high protein UIP sources. Rumen protected amino acids
may develop as a cost effective means to supplement microbial protein deficiencies in amino acid
supply to meet the requirements of high producing cows.
Additional Readings
WCDS-1995:
Barley - As a Grain
and Forage Source for Ruminants
Applied Dairy Science Course - University of Alberta:
Ruminal Digestion of
carbohydrates, protein, and lipids.
References
1. Aquire, E. O., A.L. Goetch, and F.N. Owens. 1984.
Corn grain processing and site of digestion by heifers. In: Anim. Sci. Res. Rep. MP116,
Oklahoma State Univ., Stillwater , pp 190.
2. Baird, G.B., J.G. Vander Walt, and E.N. Bergman.
1983. Whole-body metabolism of glucose and lactate in productive sheep and cows. Br. J. Nutr.
50:249.
3. Baldwin, R.L., N.E. Smith, J. Taylor and M. Sharp.
1980 Manipulating metabolic parameters to improve growth rate and milk secretion. Journal of
Animal Sci. 51:1416.
4. Baldwin, R.L. 1970. Energy metabolism in anaerobes,
Am. J. Clin. Nutr. 23:1508.
5. Bauchhop, T. and S.R. Elsden. 1960. The growth of
microorganisms in relation to their energy supply. J. Gen Microbiol. 23:457.
6. Casper, D.P., D.J. Schingoethe and W.A. Eisenbeisz.
1990. Response of early lactating dairy cows fed diets varying in source of nonstructural
carbohydrate and crude protein. J. Dairy Science. 72:1039.
7. Casper, D.P. and D.J. Schingoethe. 1989. Lactational
response of dairy cows to diets varying in ruminal solubilities of carbohydrates and crude protein.
J. Dairy Sci. 72:928.
8. Cerneau, P. and B. Michalet-Doreau. 1991. In situ
starch degradation of different feeds in the rumen. Reprod. Nutr. Dev. 31:65.
9. Cole, N.A., R.R. Johnson and F.N. Owens. 1976.
Influence of roughage level and corn processing method on the site and extent of digestion by beef
steers. J. Anim. Sci. 43:490.
10. Conrad, H.R. 1966. Symposium on factors
influencing the voluntary intake of herbage by ruminants: physiological and physical factors
limiting feed intake. J. Anim. Sci. 25: 227.
11. DePeters, E.J. and S.J. Taylor. 1985. Effects of
feeding corn or barley on composition of milk and diet digestibility. J. Dairy Sci. 68:2027.
12. Garcia, J.A., C.B. Theurer and W.H. Hale. 1981.
Digestao ruminal e pos-ruminal do amido, em novilhos alimenatados comdois niveis de grao de
sorgo floculado. Rev. Soc. Bras. Zootec. 10:235.
13. Gaylean, M.L., D.G. Wagner and F.N. Owens. 1979.
Corn particle size and site and extent of digestion by steers. J. Anim. Sci. 49:204.
14. Gaylean, M.L., D.G. Wagner and R.R. Johnson.
1976. Site and extent of starch digestion in steers fed processed corn rations. J. Anim. Sci.
43:1088.
15. Gaylean, M.L., D.G. Wagner and F.N. Owens. 1981.
Dry matter and starch disappearance of corn and sorghum as influenced by particle size and
processing. J. Dairy Sci. 64:1804.
16. Hale, W.H. 1973. Influence of processing on the
utilization of grains (starch) by ruminants. J. Anim. Sci. 37:1075.
17. Herrera-Saldena, R. and J.T. Huber. 1989. Influence
of varying protein and starch degradabilities on performance of lactating cows. J. Dairy Sci.
72:1477.
18. Hibberd, C.A., D.G. Wagner, R.L. Hintz and D.D.
Griffen. 1983. Effect of sorghum grain variety and processing method on site and extent of starch
digestion in steers. Page 28 in Anim. Sci. Res. Rep. MP114, Oklahoma State Univ.,
Stillwater.
19. Hinman, D.D. and R.R. Johnson. 1974. Influence of
degree of micronization on the site and extent of sorghum starch digestion in beef cattle fed high
concentrate rations. J. Anim. Sci. 39:958.
20. Holmes, J.H.G., M.J. Drennan and W.N. Garrett.
1970. Digestion of steam-processed milo by ruminants. J. Anim. Sci. 31:409.
21. Isaacson, H.R., F.C. Hinds, M.P. Bryant and F.N.
Owens. 1975. Efficiency of energy utilization by mixed rumen bacteria in continuous culture. J.
Dairy Sci. 58:1645.
22. Janes, A.N., T.E.C. Weekes and D.G. Armstrong.
1985. Absorption and metabolism of glucose by the mesenteric-drained viscera of sheep fed on
dried-grass or ground, maize-based diets. Br. J. Nutr. 54:449.
23. Kartchner, R.J. 1972. Starch digestion in the bovine
as influenced by level and processing of sorghum grain. M.S. Thesis, Univ. Arizona, Tucson.
24. Klusmeyer, T.H., G.L. Lynch and J.H. Clark. 1991.
Effects of calcium salts of fatty acids and proportion of forage in diet on ruminal fermentation and
nutrient flow to duodenum of cows. J. Dairy Sci. 74:2220.
25. Klusmeyer, T.H., G.L. Lynch and J.H. Clark. 1990.
Effects of source and amount of protein on ruminal fermentation and passage of nutrients to the
small intestine of lactating cows. J. Dairy Sci.
26. Kronfeld, D.S., G.P. Mayer, J.M. Robertson and F.
Raggi. 1963. Depression of milk secretion during insulin administration. J. Dairy Sci. 46:553.
27. Kronfeld, D.S. 1976. The potential importance of the
proportions of glucogenic, lipogenic and aminogenic nutrients in regard to the health and
productivity of dairy cows. Adv. Anim. Physiol. Anim. Nutr. 7:5.
28. Kronfeld, D.S., Fl Raggi and C.F. Ramberg. 1968.
Mammary blood flow and ketone metabolism is normal, fasted and ketotic cows. Am. J. Phisiol.
215:218.
29. Lee, R. W., M.L. Galyean and G.P. Lofgreen. 1982.
Effects of mixing whole shelled and steamflaked corn in finishing diets on feedlot performance
and site and extent of digestion in beef steers. J. Anim. Sci. 55:475.
30. McCarthy, R.D., T.H. Klusmeyer, J.L. Vicini, J.H.
Clark and D.R. Nelson. 1989. Effect of source of protein and carbohydrate on ruminal
fermentation and passage of nutrients to the small intestine of lactating cows. J. Dairy Sci.
72:2002.
31. McNeil, J.W., G.D. Potter and J.K. Riggs. 1971.
Ruminal and postruminal carbohydrate utilization in steers fed processed sorghum grain. J. Anim.
Sci. 33:1371.
32. Metwally, H.M. 1989. Effects of dietary
carbohydrate and protein supply to the small intestine. Ph.D. Diss., Univer. Minnesota, St. Paul.
33. Moe, P. W. and H.F. Tyrrell. 1976. Estimating
metabolizable and net energy of feeds. Proc. 1st Int. Symp. Feed Composition Anim. Nutrient
Requirements Computerization Diets. Int. Feedstuffs Inst., Logan, UT.
34. Moran, J.B. 1986. Cereal grains in complete diets for
dairy cows: a comparison of rolled barley, wheat and oats and of three methods of processing oats.
Anim. Prod. 43:217.
35. Morgan, D. A., H. Ryan and P.K. Upton. 1989.
Cereal digestion in the ruminant. 1. Nutrient passage from the rumen and glucose kinetics in
sheep fed unground barley in ungrounded maize diets. Ir. J. Agric. Res. 28:35.
36. Nocek, J.E. 1987. Characterization of in situ dry
matter and nitrogen of various corn grain forms. J. Dairy Sci. 70:2291.
37. Nocek, J.E. and J.B. Russell. 1988. Protein and
energy as an integrated system. Relationship of ruminal protein and carbohydrates availability to
microbial synthesis and milk production. J. Dairy Sci. 71:2070.
38. Nocek, J.E. and S. Tamminga. 1991. Site of
digestion of starch in the gastrointestinal tract of dairy cows and its effect on milk yield and
composition. J. Dairy Sci. 74:3598.
39. Oliveria, J., J.T. Huber, D. Ben-Ghedalia and M.
Pessarakli. 1990. Effect of sorghum grain processing on the performance of lactating dairy cows.
J. Dairy Sci. (Suppl. 1) 73:127. (Abstr.).
40. Owens, FlN., R.A. Zinn and Y.K. Kim. 1986. Limits
to starch digestion in the ruminants small intestine. J. Anim. Sci. 63:1634.
41. Pethick, D.W., D.B. Lindsay, P.J. Barker and R. T.
Northrup. 1981. Acetate supply and utilization by tissues of sheep in vivo. Br. J. Nutr. 46:97.
42. Pirt, S.J. 1965. The maintenance energy of bacteria
in growing cultures. Proc. R. Soc. London Ser. B. 163:224.
43. Poo re, M.H., J.A. Moore, R.S. Swingle, W.H.
Brown and F.M. Whiting. 1989. Influence of forage quality and sorghum grain processing in diets
formulated to contain 25% forage NDF on milk production by Holstein cows. J. Dairy Sci. (Suppl.
1) 72:480 (Abstr.).
44. Reynolds, C.K., G.B. Huntington, H.F. Tyrrell and
P.J. Reynolds. 1988. Net protal-drained visceral and hepatic metabolism of glucose, Llactate, and
nitrogenous compounds in lactating Holstein cows. J. Dairy Sci. 71:1803.
45. Reynolds, C.K. and G.B. Huntington. 1988. Partition
of portal-drained visceral net flux in beef steers. 2. Net flux of volatile fatty acids, Dbhydroxy
butyrate and L-lactate across stomach and post-stomach tissues. Br. J. Nutr. 60:553.
46. Reynolds, C.K. and G.B. Huntington. 1988. Partition
of portal-drained visceral net flux in beef steers 1. Blood flow and net flux of oxygen, glucose and
nitrogenous compounds across stomach and post-stomach tissues. Br. J. Nutr. 60:539.
47. Reynolds, C.K., H.F. Tyrrell and P.J. Reynolds.
1991. Effects of diet forage-to-concentrate ratio and intake on energy metabolism in growing beef
heifers: whole body energy and nitrogen balance and visceral heat production. J. Nutr. 121:994.
48. Robinson, P.H. and J.J. Kennelly. 1989. Influence of
high moisture barley on digestibility, kinetics of rumen ingesta turnover, and milk production in
dairy cows. Can. J. Anim. Sci. 69:195.
49. Rooney, L.W. and R.L. Pfugfelder. 1986. Factors
affecting starch digestibility with special emphasis on sorghum and corn. J. Anim. Sci.
63:1607.
50. Russell, J.B. 1984. Factors influencing competition
and composition of rumen bacterial flora. In: Gilchrist, F.M.C., Machie, R.I. eds. Proc, Symp. on
Herbiovore Nutr. in Sub-tropics and. Craighall, So. Africa: The Sci. Press p. 313-345.
51. Russell, J.B. and R.L. Baldwin. 1979. Comparison
of maintenance energy expenditures and growth yields among several rumen bacteria grown on
continuous culture. Appl. Environ. Microbiol. 37:537.
52. Russell, J.B. 1986. Ecology of rumen
microorganisms: Energy use in aspects of digestive physiology in ruminants. Ed. Dobson &
Dobson. Comstock Publ. Assoc.
53. Schuldt, A. 1989. Enfluss der Getreideart, der
technischen Behandlung von Getreide sowie der Rationzusammensetzung auf Ort and Usmass der
Verdauung der Getreidestarke in Milchkuhen. Ph.D. Diss. Christian Albrechts Univ., Kiel,
Germany.
54. Spicer, L.A, C.B. Theurer, J. Souse and T.H. Noon.
1986. Ruminal and postruminal utilization of nitrogen and starch from sorghum grain, corn, and
barley based diets by beef steers. J. Anim. Sci. 62:521.
55. Stokes, S.R. 1990. The effects of different
carbohydrate and protein types on ruminal fermentation and microbial metabolism. Ph.D. Diss.,
West Virginia Univ., Morgantown.
56. Sutton, J.D. 1985. Digestion and absorption of
energy substrates in the lactating cow. J. Dairy Sci. 68:3376.
57. Swingle, R.S., J. Moore, M. Poore and T. Eck. 1990.
Utilization of starch from processed sorghum grain. Page 52 in Proc. Southwestern Nutr. Manage.
Conf. Feb. 1-2, Tempe, AZ.
58. Tamminga, S., P. Van der Togt, C.J. Van der
Koelen, C. Melieftste, M. Luttikuis and G.D.H. Claassen. 1989. The behavior of starch in the
rumen of dairy cows. Mededeling No. 14. Inst. Livest. Feeding Nutr. Lelystad, Neth.
59. Tamminga, S., M.A. Van Vuuren, C.J. Van der
Koelen, R.S. Ketelaar and P.L. Van der Togt. 1990. Ruminal behavior of structural carbohydrates,
non-structural carbohydrates and crude protein from concentrate ingredients in dairy cows. Neth J.
Agric. Sci. 38:513.
60. Tempest, D.W. and O.M. Neijssel. 1984. The status
of ATP and maintenance energy as biologically interpretable phenomena. Ann. Rev. Microbiol.
38:459.
61. Theurer, C.B. 1986. Grain processing effects on
starch utilization by ruminants. J. Anim. Sci. 63:1649.
62. Thorne, M.J., L.J. Thompson and D.J.A. Jenkins.
1983. Factors affecting starch digestibility and the glycemic response with special reference to
legumes. Am. Clin. J. Nutr. 38:481.
63. Van der Walt, J.G., G.D. Baird and E.N. Bergman.
1983. Tissue glucose and lactate metabolism and interconversions in pregnant and lactating
sheep. Br. J. Nutr. 50:267.
64. Vearsilerp, T. 1986. Site and extent of maize starch
digestion in relation to stage of maize maturity in lactating cows. Ph.D. Diss., Christian Albrecht
Univ., Kiel, Germany.
65. Voight, J., B. Piatkowski, R. Krawielitzki and K.O.
Trautmann, 1977. Die Wirkung der physikalischen Form von Trockengrunfutter and
verschiedener Starkequlllen auf den Ort der Verdgauung der Kohlenhydrate and des Proteins
sowie die bakterielle Proteinsynthese im Pansen der Kuh. Archiv. Tierernaehr. 27:393.
66. Voight, J., B. Piatkowski, S. Nagel and R.
Krawielitzki. 1976. Einfluss der physikalischen Form von Weizenstroh and verschiedener
Starkequellen auf den Ort der Verdauung von starke and Rohzellulose bei der Kuh. Archiv.
Tiercernaehr. 26:345.
67. Voight, J., B. Piatkowski and R. Krawielitzki. 1978.
Die Wirkung der Reihenfolge vo Grobbfutter and Konzentraten in der Futterung auf die
Kohlenhydratverdauung and die bakterielle Proteinsynthese im Pansen der Milchkuh. Archiv.
Tierernaehr. 28:67.
68. Watson, M. J., G.P. Savage, I. Brown and D.G.
Armstrong. 1972. Sites of disappearance of apparently digestible cellulose and apparently
digestible linked glucose polymers in the digestive tract of a cow receiving dried grass-concentrate
diets. Proc. Nutr. Soc. 31:991.
69. Zinn, R.A. 1990. Influence of flake density on the
comparative feeding value of steam-flaked corn for feedlot cattle. J. Anim. Sci. 68:767.